4. How the Hortens Design Their
Aircraft
Introduction
The Hortens started their
careers as aircraft designers in a very practical way, without assistance
from highbrow theory. Early designs were based mainly on what they
found satisfactory on a small-scale model. As time went on Reimar
Horten began theoretical investigations of various problems that took his
fancy and built up a fairly complex basic design procedure. Some
of his methods seem strange to us and some important aspects he still leaves
to “experience” where we tend to trust theory. The following is a
brief account of his methods as related to us at Gottingen in September
1945.
4.1 Wing Section Design
Wing
sections were designed from scratch and were never wind tunnel tested.
The only exception to this rule was the disastrous adoption of the Mustang
profile for the H IVb and the H XII.
Camber
lines were designed by Birnbaum’s thin aerofoil theory to give zero Cmo.
This gives an equation for the case of 3% maximum camber:
0.03
y = 0.1055 x (1 – x)3
dy d2y
This has dx and dx2 both
zero at x = 1 and gives y = max at x = 0.25
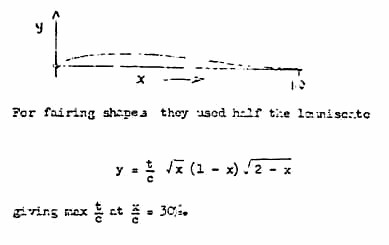
For fairing
shapes with maximum thickness at 40% they used a geometrical projection
method due to Ringleb.
To
get good stalling characteristics the following criterion was used:
p/c
(t/c)2 = 1.0
Where p = nose
radius
c = chord
t = maximum thickness
This criterion is well
known and a report by Kawalki of D.V.L. has been published on the subject.
Wing tip sections
are made symmetrical because Horten dislikes the idea of a cambered section
with negative flap deflection at the stall.
Horten thought
that position of the maximum thickness of the wing section had a definite
influence on the sweepback that could be used (and vice-versa) due to the
influence on lateral flow in the boundary layer. He suggested that
following rough rule for 12% thick sections
Max Thickness Maximum Sweep
Location
(Leading Edge)
30%
45°
40%
35°
50%
20°
This rule was based
on his experience of the flying qualities of aircraft so far built.
4.2 Calculation of Aerodynamic Centers
Aerodynamic
center was calculated by integration of the product of local loading x
distance of the local aerodynamic center behind a convenient spanwise datum.
Load distribution was first calculated by Weissingers method for a sweptback
wing. Details of this were not known but it was apparently a development
of Multopp’s method which extended the lifting line theory to take account
of chordwise pressure distribution and the influence of this on induced
velocity along the span. Load distribution was used to give values
of
d CL local
da wing.
Local aerodynamic center was assumed to be at
0.25 x n x C from the leading edge, n being a factor representing the departure
of the two-dimensional lift curve slope from 2 pi.
n had approximately the following values for different thickness ratios:
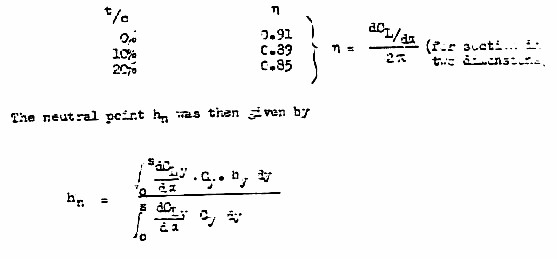
Center of gravity positions
were specified by Horten as distances ahead of the above neutral point
in terms of a dimension called the “Pfeilmass”. The Pfeilmass is
a measure of the fore and aft dimension of the wing and is defined by:
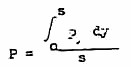
(ed. – top line of equation is Py dy.)
Py is the fore and aft distance between
the aerodynamic center of the center section and the aerodynamic center
at the general point y.
4.3 Fixing the Layout
Preliminary Determination of CG Position
As a first approximation
Horten used the following graphical contstruction to give a mean chord
and mean quarter chord point.
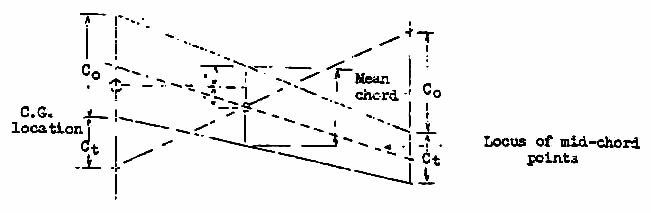
The first approximation
to the CG position was taken as the man quarter chord point defined as
above.
It will be noticed
that the mean chord used by Horten is the local chord line passing through
the center of area of the half wing. The above construction does
not apply to planforms differing greatly from a trapezium. The chord
length so defined is not the same as that given by s / b.
Wing Twist
The procedure here was
to construct curves from which static margin could be chosen if wing twist
had been decided, or, more usually, to choose twist for a given static
margin, assuming in either case that the desired CL with elevons
neutral was known.
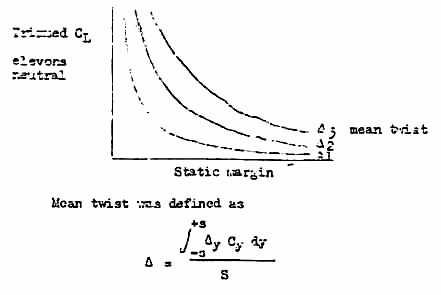
Where Delta = mean twist
Deltay = twist at general point y
Cy = chord at general point y
S = wing area
s = semi span
Desirable static margins
were known from experience and Horten gave the following table (all in
% of Pfeilmass) of values for different Horten aircraft.
Static Margin
Type
(% p)
Comments
II
5%
III
4%
Normal position
2%
Minimum for satisfactory longitudinal stability
5%
For best longitudinal characteristics
3%
For optimum performance
IV
5%
Normal
6-7%
Best Handling
V, VII, IX 2-3%
On
sailplanes, twist was designed to give elevon neutral trim near to the
CL for best gliding angle and on power aircraft trim at cruising
CL. Center section head fairing were found to have an
appreciable effect on trim.
In
the case of high speed aircraft, selection of mean twist was farther complicated
by the need to avoid local shock stall at high speed. The method
of dealing with this was described in para. 3.11 under “Aerodynamic Design”.
Twist
distribution was determined by the type of aircraft. For a sailplane,
which spends much of its time in circling flight, Horten had developed
a theory which enabled twist distribution to be designed so that the glider
was in trim laterally and directionally without elevon or rudder deflection.
In the calculation the twist needed to give static equilibrium was found,
taking into account variation of incidence, speed, profile and induced
drag across the span and assuming straight trailing vortices.
The answer was a twist
of the form
y y
e = eo ( A s + B ( s )3 )
(ed. – missing text about one of the terms
being indeterminate.)
On the H IV for example, twist was designed to give trim in a 45° banked
turn at CL = 1. Incidence difference between the tips
was 1° and the twist was
y ( y)2 (
y)3
e = 2° ( s + s +
s )
An additional aerodynamic
twist of 1.1° was added giving an overall designed washout of 7.1°.
The second power term was introduced to satisfy the condition for longitudinal
trim (flaps neutral for trimmed flight at 100 kph on the H IV and 140 kph
on the H VI).
On the H III the linear
term was much bigger (4°) and the incidence difference between the
tips 6° in the specified circling condition.
Torsional deflection
of the wing was allowed for in these calculations.
In addition to the above
requirements Horten also designs the combination of taper and twist to
ensure that local stalling lift coefficient is first reached at the middle
third of the semi span. Apparently all those conditions can be satisfied
simultaneously, the linear term was said to be available mainly for stall
control whilst the cubic term gave the required rolling equilibrium.
Sweepback
Sweepback
is governed to some extent by the load being carried, but for low speed
aircraft Horten liked to keep leading edge sweepback below 45° to avoid
loss of controller power through boundary layer outflow. For high
speed aircraft, high sweepback was an advantage, for besides keeping drag
down it prevented over sensitivity of control.
4.4 Control Design
His calculations
of control forces were customary, design was governed by experience.
Aileron performance was however calculated on the H IX.
The change over
from round nosed to Frise nosed controls was made to improve the yawing
characteristics with aileron applications. The subdivision of the
flap into two parts enabled differential to be used to improve the favorable
yaw with aileron. The outer Frise nose in this case balanced the
round nosed inner flap also. In the three stage flap, where the outer
flap behaved principally as up going aileron, it was possible to alter
the relative balance between aileron and elevator (the latter being usually
too light relative to the aileron) and produce better harmony of control.
This was aid to be especially important in high aspect ratio sailplanes
(or airplanes ling the H VIII) where the ratio of lateral inertia
to longitudinal inertia is high (e.g. this ratio was about 30 on the
H VI compared with 5 on the H IX). Further questioning revealed that
Horten thought lateral inertia important because the initial response (acceleration)
when correcting small gust disturbances depended largely on inertia although
the final rate of roll was hardly affected.
Drag rudder design
was evolved entirely by flight experiment with no wing tunnel data to help.
4.5 Flight Stability
Dynamic stability
was never investigated theoretically and was not studied very carefully
in flight. Reliance was placed mainly on general impressions of the
pilot and we found no evidence of results having been analyzed critically.
The Hortens were obviously
not in the habit of thinking in terms of periods and dampings, and Reimar
did not know that lv and nv were for his various designs; dihedral was
fixed by experience.
The “stick force
per g” criterion was not used and although elevator angles to trim were
considered in the design stage there was no methodical flight check.
4.6 Undercarriage Design
During the construction
of their series of aircraft the Hortens had been forced to try a number
of unorthodox undercarriage layouts using 2, 3 and 4 wheels. The
tricycle and four wheel layouts used wheel positions giving a wide range
of weight distribution. The following figures were quoted:
Type
H IV
H V
H VIII
Nose Wheel Reaction
8
55
15
(% A.U.W.)
Main Wheel Reaction
92
45
85
The H VII and H IX also
take a large proportion of the weight on the nosewheel – of the order 40-50%.
These heavy nosewheel reactions were combined with large ground incidence
to enable the aircraft to fly off the ground.
According to Horten
none of the layouts tested had given any trouble due to porporsing or instability
to unstick; he was inclined to dismiss undercarriage design as presenting
no problems.
4.7 Stressing
Horten stated
that there were no special requirements for stress calculations on tailless
aircraft. The H IX was designed for a normal acceleration (n) of
7g combined with a safety factor (j) of 1.8. Other design considerations
were as follows:
(a) Gusts of + 10 m/sec. in a dive at 1100
kph with j = 1.2. The air was assumed incompressible for this calculation
except that dCL / da was arbitrarily increased 50%
over the incompressible value. A relieving factor of 0.6 was applied.
(b) A complete aileron roll (360°) was
to be possible at 900 kph at 2500 m. in 4 seconds, including allowance
for aero elastic distortion. This was both a performance and a stressing
requirement.
(c) There were no official aileron reversal
requirements but Hortens designed the H IX for a reversal speed of 1.2
x diving speed (1320 kph) assuming incompressible flow.
A peculiar feature
in the structural design of the H VII was mentioned. It was stated
that the calculated change of trim to cause a 4g dive pull out at diving
speed was only 0.3° of elevon, when allowance was made for aero elastic
distortion. This was improved by increasing the ply skin thickness
from 1.5 mm to 2.5 mm. The phenomenon would be more understandable
if the torsion component of spar bending had been large but Horten says
that this was not included.
Actual figures
quoted were:
Elevator Angle
1.5 mm Ply 2.5 mm Ply
Dive
+3°
+2.5°
4g Pullout
+2.7°
-0.5°
..PREVIOUS
SECTION.............................. ..NEXT
SECTION
|